Protective effects of astragaloside IV against hypertension-induced vascular remodeling involves the DNMT1 and TET2 signaling pathway
DOI:
https://doi.org/10.2298/ABS210426024QKeywords:
hypertension, vascular remodeling, vascular smooth muscle cells (VSMC), astragaloside IV, DNA methyltransferase1 (DNMT1), ten-eleven translocation 2 (TET2)Abstract
Paper description:
- Astragaloside IV (AS-IV), a triterpene saponin from Astragalus membranaceus, has a beneficial effect in cardiovascular disease.
- In two-kidney one-clip (2K1C) hypertensive rats, AS-IV: reduced blood pressure; reversed hypertension-induced thoracic media thickness; inhibited DNMT1, MMP2, PCNA; increased SM22α, ACTA2, TET2 expression in the aorta; in vitro it inhibited the proliferation and migration in VSMCs treated with angiotensin II; induced SM22α, ACTA2, TET2; reduced COL-1a, COL-3a, DNMT1, MMP2, PCNA expression; modulated 5-methylcytosine expression.
- AS-IV reversed hypertension-induced vascular remodeling by inhibiting DNMT1, upregulating TET2.
- Novel insight for As-IV treatment of vascular disease is provided.
Abstract: Proliferation, migration, and the phenotypic switch of vascular smooth muscle cells (VSMCs) play an important role in vascular remodeling induced by hypertension. Astragaloside IV (AS-IV), the active ingredient of Astragalus membranaceus, has been shown to exert a beneficial role in cardiovascular disease. The present study aimed to investigate the mechanism responsible for the protective effects of AS-IV on hypertension-induced vascular remodeling. AS-IV significantly reduced blood pressure and aortic media thickness in two-kidney, one-clip (2K1C) hypertensive rats. AS-IV administration downregulated the expression levels of DNA methyltransferase1 (DNMT1), matrix metalloproteinase (MMP2) and proliferating cell nuclear antigen (PCNA) and upregulated the expression of smooth muscle 22α protein (SM22α), α-smooth muscle actin (ACTA2) and ten-eleven translocation 2 (TET2) in the aorta of hypertensive rats. AS-IV inhibited the proliferation and migration in VSMCs treated with angiotensin II (Ang II). AS-IV increased the expression of SM22α, ACTA2 and TET2, and decreased the expression of collagen Ia (COL-1a), collagen IIIa (COL-3a), DNMT1, MMP2 and PCNA in vitro. Reduction in 5-methylcytosine (5-mC) was observed in VSMCs treated with AS-IV. Knockdown of DNMT1 induced the expression of TET2, while the level of DNMT1 did not change after knockdown of TET2. These results suggest that AS-IV reversed hypertension-induced vascular remodeling by inhibiting DNMT1 and upregulating TET2.
Downloads
References
Lackland DT, Weber MA. Global burden of cardiovascular disease and stroke: Hypertension at the core. Can J Cardiol. 2015;31:569-71.
Mills KT, Bundy JD, Kelly TN, Reed JE, Kearney PM, Reynolds K, Chen J, He J. Global disparities of hypertension prevalence and control: A systematic analysis of Population-Based studies from 90 countries. Circulation. 2016;134:441-50. https://doi.org/10.1161/circulationaha.115.018912
Zhuge Y, Zhang J, Qian F, Wen Z, Niu C, Xu K, Ji H, Rong X, Chu M, Jia C. Role of smooth muscle cells in Cardiovascular Disease. Int J Biol Sci 2020;16:2741-51. https://doi.org/10.7150/ijbs.49871
Simon PH, Sylvestre MP, Tremblay J, Hamet P. Key considerations and methods in the study of Gene-Environment interactions. Am J Hypertens. 2016;29:891-9. https://doi.org/10.1093/ajh/hpw021
Levy E, Spahis S, Bigras JL, Delvin E, Borys JM. The epigenetic machinery in vascular dysfunction and hypertension. Curr Hypertens Rep. 2017;19:52. https://doi.org/10.1007/s11906-017-0745-y
Jones PA. Functions of DNA methylation. Islands, start sites, gene bodies and beyond. Nat Rev Genet. 2012;13:484-92. https://doi.org/10.1038/nrg3230
Meissner A, Mikkelsen TS, Gu H, Wernig M, Hanna J, Sivachenko A, Zhang X, Bernstein BE, Nusbaum C, Jaffe DB, Gnirke A, Jaenisch R, Lander ES. Genome-scale DNA methylation maps of pluripotent and differentiated cells. Nature. 2008;454:766-70. https://doi.org/10.1038/nature07107
Lee DY, Chiu JJ. Atherosclerosis and flow. roles of epigenetic modulation in vascular endothelium. J Biomed Sci. 2019;26(1):56.
Lee DY, Chiu JJ. Atherosclerosis and flow: Roles of epigenetic modulation in vascular endothelium. J Biomed Sci. 2019;26:56.
Karpe PA, Tikoo K. Heat shock prevents insulin resistance-induced vascular complications by augmenting angiotensin-(1-7) signaling. Diabetes. 2014;63:1124-39. https://doi.org/10.2337/db13-1267
Li Q, Wu J, Xu Y, Liu L, Xie J. Role of RASEF hypermethylation in cigarette smoke-induced pulmonary arterial smooth muscle remodeling. Respir Res 2019;20:52. https://doi.org/10.1186/s12931-019-1014-1
Xu L, Hao H, Hao Y, Wei G, Li G, Ma P, Xu L, Ding N, Ma S, Chen AF, Jiang Y. Aberrant MFN2 transcription facilitates homocysteine-induced VSMCs proliferation via the increased binding of c-Myc to DNMT1 in atherosclerosis. J Cell Mol Med. 2019;23:4611-26. https://doi.org/10.1111/jcmm.14341
Zhuang J, Luan P, Li H, Wang K, Zhang P, Xu Y, Peng W. The Yin-Yang dynamics of DNA methylation is the key regulator for smooth muscle cell phenotype switch and vascular remodeling. Arterioscler Thromb Vasc Biol. 2017;37:84-97. https://doi.org/10.1161/atvbaha.116.307923
Kohli RM, Zhang Y. TET enzymes, TDG and the dynamics of DNA demethylation. Nature. 2013;502:472-9. https://doi.org/10.1038/nature12750
Ito S, Shen L, Dai Q, Wu SC, Collins LB, Swenberg JA, He C, Zhang Y. Tet proteins can convert 5-methylcytosine to 5-formylcytosine and 5-carboxylcytosine. Science (New York, N.Y.) 2011;333:1300-3. https://doi.org/10.1126/science.1210597
Aavik E, Babu M, Yla-Herttuala S. DNA methylation processes in atheosclerotic plaque. Atherosclerosis. 2019;281:168-79. https://doi.org/10.1016/j.atherosclerosis.2018.12.006
Joshi SR, Kitagawa A, Jacob C, Hashimoto R, Dhagia V, Ramesh A, Zheng C, Zhang H, Jordan A, Waddell I, Leopold J, Hu CJ, McMurtry IF, D'Alessandro A, Stenmark KR, Gupte SA. Hypoxic activation of glucose-6-phosphate dehydrogenase controls the expression of genes involved in the pathogenesis of pulmonary hypertension through the regulation of DNA methylation.Am J Physiol Lung Cell Mol Physiol. 2020;318:L773-86. https://doi.org/10.1152/ajplung.00001.2020
Liu R, Jin Y, Tang WH, Qin L, Zhang X, Tellides G, Hwa J, Yu J, Martin KA. Ten-eleven translocation-2 (TET2) is a master regulator of smooth muscle cell plasticity. Circulation. 2013;128:2047-57. https://doi.org/10.1161/circulationaha.113.002887
Li B, Zang G, Zhong W, Chen R, Zhang Y, Yang P, Yan J. Activation of CD137 signaling promotes neointimal formation by attenuating TET2 and transferring from endothelial cell-derived exosomes to vascular smooth muscle cells. Biomed Pharmacother. 2020;121:109593. https://doi.org/10.1016/j.biopha.2019.109593
Zeng Z, Xia L, Fan S, Zheng J, Qin J, Fan X, Liu Y, Tao J, Liu Y, Li K, Ling Z, Bu Y, Martin KA, Hwa J, Liu R, Tang WH. Circular RNA CircMAP3K5 acts as a MicroRNA-22-3p sponge to promote resolution of intimal hyperplasia via TET2-Mediated smooth muscle cell differentiation. Circulation. 2021;143:354-71. https://doi.org/10.1161/circulationaha.120.049715
Fu J, Wang Z, Huang L, Zheng S, Wang D, Chen S, Zhang H, Yang S. Review of the botanical characteristics, phytochemistry, and pharmacology of Astragalus membranaceus (Huangqi). Phytother Res. 2014;28:1275-83. https://doi.org/10.1002/ptr.5188
Zhang J, Wu C, Gao L, Du G, Qin X. Astragaloside IV derived from Astragalus membranaceus: A research review on the pharmacological effects. Adv Pharmacol. 2020;87:89-112. https://doi.org/10.1016/bs.apha.2019.08.002
Zhang DQ, Li JS, Zhang YM, Gao F, Dai RZ. Astragaloside IV inhibits Angiotensin II-stimulated proliferation of rat vascular smooth muscle cells via the regulation of CDK2 activity. Life Sci. 2018;200:105-9. https://doi.org/10.1016/j.lfs.2018.03.036
Yuan W, Zhang Y, Ge Y, Yan M, Kuang R, Zheng X. Astragaloside IV inhibits proliferation and promotes apoptosis in rat vascular smooth muscle cells under high glucose concentration in vitro. Planta Med. 2008;74:1259-64. https://doi.org/10.1055/s-2008-1081290
Yao J, Fang X, Zhang C, Yang Y, Wang D, Chen Q, Zhong G. Astragaloside IV attenuates hypoxiainduced pulmonary vascular remodeling via the Notch signaling pathway. Mol Med Rep. 2021;23:1. https://doi.org/10.3892/mmr.2020.11726
Jin H, Jiao Y, Guo L, Ma Y, Zhao R, Li X, Shen L, Zhou Z, Kim SC, Liu J. Astragaloside IV blocks monocrotaline‑induced pulmonary arterial hypertension by improving inflammation and pulmonary artery remodeling. Int J Mol Med. 2021;47:595-606. https://doi.org/10.3892/ijmm.2020.4813
Zhang WD, Zhang C, Wang XH, Gao PJ, Zhu DL, Chen H, Liu RH, Li HL. Astragaloside IV dilates aortic vessels from normal and spontaneously hypertensive rats through endothelium-dependent and endothelium-independent ways. Planta Med. 2006;72:621-6. https://doi.org/10.1055/s-2006-931572
Lee SH, Lee YH, Jung SW, Kim DJ, Park SH, Song SJ, Jeong KH, Moon JY, Ihm CG, Lee TW, Kim JS, Sohn IS, Lee SY, Kim DO, Kim YG. Sex-related differences in the intratubular renin-angiotensin system in two-kidney, one-clip hypertensive rats. Am J Physiol Renal Physiol. 2019;317:F670-82. https://doi.org/10.1152/ajprenal.00451.2018
Martins-Oliveira A, Castro MM, Oliveira DM, Rizzi E, Ceron CS, Guimaraes D, Reis RI, Costa-Neto CM, Casarini DE, Ribeiro AA, Gerlach RF, Tanus-Santos JE. Contrasting effects of aliskiren versus losartan on hypertensive vascular remodeling. Int J Cardiol. 2013;167:1199-205. https://doi.org/10.1016/j.ijcard.2012.03.137
Tan J, Xie Y, Yao A, Qin Y, Li L, Shen L, Zhang X, Xu C, Jiang X, Wang A, Yan Z. Long noncoding RNA-dependent regulation of vascular smooth muscle cell proliferation and migration in hypertension. Int J Biochem Cell Biol. 2020;118:105653. https://doi.org/10.1016/j.biocel.2019.105653
Pernomian L, Do PA, Silva BR, de Paula TD, Grando MD, Bendhack LM. C-type natriuretic peptide-induced relaxation through cGMP-dependent protein kinase and SERCA activation is impaired in two kidney-one clip rat aorta. Life Sci. 2021;272:119223. https://doi.org/10.1016/j.lfs.2021.119223
Yang C, Wu X, Shen Y, Liu C, Kong X, Li P. Alamandine attenuates angiotensin II-induced vascular fibrosis via inhibiting p38 MAPK pathway. Eur J Pharmacol. 2020;883:173384. https://doi.org/10.1016/j.ejphar.2020.173384
Jiang P, Ma D, Wang X, Wang Y, Bi Y, Yang J, Wang X, Li X. Astragaloside IV prevents Obesity-Associated hypertension by improving Pro-Inflammatory reaction and leptin resistance. Mol Cells. 2018;41:244-55.
Sun Y, Lu M, Sun T, Wang H. Astragaloside IV attenuates inflammatory response mediated by NLRP-3/calpain-1 is involved in the development of pulmonary hypertension. J Cell Mol Med. 2021;25:586-90. https://doi.org/10.1111/jcmm.15671
Zhang X, Chen J, Xu P, Tian X. Protective effects of astragaloside IV against hypoxic pulmonary hypertension. Medchemcomm. 2018;9:1715-21. https://doi.org/10.1039/c8md00341f
Lin XP, Cui HJ, Yang AL, Luo JK, Tang T. Astragaloside IV improves vasodilatation function by regulating the PI3K/Akt/eNOS signaling pathway in rat aorta endothelial cells. J Vasc Res. 2018;55:169-76. https://doi.org/10.1159/000489958
Zhang C, Wang XH, Zhong MF, Liu RH, Li HL, Zhang WD, Chen H. Mechanisms underlying vasorelaxant action of astragaloside IV in isolated rat aortic rings. Clin Exp Pharmacol Physiol. 2007;34:387-92. https://doi.org/10.1111/j.1440-1681.2007.04564.x
Chen Z, Cai Y, Zhang W, Liu X, Liu S. Astragaloside IV inhibits platelet-derived growth factor-BB-stimulated proliferation and migration of vascular smooth muscle cells via the inhibition of p38 MAPK signaling. Exp Ther Med. 2014;8:1253-8. https://doi.org/10.3892/etm.2014.1905
Mohammad G, Kowluru RA. Homocysteine disrupts balance between MMP-9 and its tissue inhibitor in diabetic retinopathy: The role of DNA methylation. Int J Mol Sci. 2020;21(5):1771. https://doi.org/10.3390/ijms21051771
Chen J, Zhang J, Wu J, Zhang S, Liang Y, Zhou B, Wu P, Wei D. Low shear stress induced vascular endothelial cell pyroptosis by TET2/SDHB/ROS pathway. Free Radic Biol Med. 2021;162:582-91. https://doi.org/10.1016/j.freeradbiomed.2020.11.017
Yu Y, Yan R, Chen X, Sun T, Yan J. Paeonol suppresses the effect of ox-LDL on mice vascular endothelial cells by regulating miR-338-3p/TET2 axis in atherosclerosis. Mol Cell Biochem. 2020;475:127-35. https://doi.org/10.1007/s11010-020-03865-w
Zhong W, Li B, Xu Y, Yang P, Chen R, Wang Z, Shao C, Song J, Yan J. Hypermethylation of the Micro-RNA 145 promoter is the key regulator for NLRP3 Inflammasome-Induced activation and plaque formation. JACC Basic Transl Sci. 2018;3:604-24. https://doi.org/10.1016/j.jacbts.2018.06.004
Potus F, Pauciulo MW, Cook EK, Zhu N, Hsieh A, Welch CL, Shen Y, Tian L, Lima P, Mewburn J, D'Arsigny CL, Lutz KA, Coleman AW, Damico R, Snetsinger B, Martin AY, Hassoun PM, Nichols WC, Chung WK, Rauh MJ, Archer SL. Novel mutations and decreased expression of the epigenetic regulator TET2 in pulmonary arterial hypertension. Circulation. 2020;141:1986-2000. https://doi.org/10.1161/circulationaha.119.044320
Belo VA, Guimaraes DA, Castro MM. Matrix metalloproteinase 2 as a potential mediator of vascular smooth muscle cell migration and chronic vascular remodeling in hypertension. J Vasc Res. 2015;52:221-31. https://doi.org/10.1159/000441621
Kopaliani I, Martin M, Zatschler B, Bortlik K, Muller B, Deussen A. Cell-specific and endothelium-dependent regulations of matrix metalloproteinase-2 in rat aorta. Basic Res Cardiol. 2014;109:419. https://doi.org/10.1007/s00395-014-0419-8
Blascke De Mello MM, Parente JM, Schulz R, Castro MM. Matrix metalloproteinase (MMP)-2 activation by oxidative stress decreases aortic calponin-1 levels during hypertrophic remodeling in early hypertension. Vascul Pharmacol. 2019;116:36-44. https://doi.org/10.1016/j.vph.2018.10.002
Seo KW, Lee SJ, Kim YH, Bae JU, Park SY, Bae SS, Kim CD. Mechanical stretch increases MMP-2 production in vascular smooth muscle cells via activation of PDGFR-β/Akt signaling pathway. Plos One. 2013;8:e70437. https://doi.org/10.1371/journal.pone.0070437
Castro MM, Rizzi E, Figueiredo-Lopes L, Fernandes K, Bendhack LM, Pitol DL, Gerlach RF, Tanus-Santos JE. Metalloproteinase inhibition ameliorates hypertension and prevents vascular dysfunction and remodeling in renovascular hypertensive rats. Atherosclerosis. 2008;198:320-31. https://doi.org/10.1016/j.atherosclerosis.2007.10.011
Newby AC. Matrix metalloproteinases regulate migration, proliferation, and death of vascular smooth muscle cells by degrading matrix and non-matrix substrates. Cardiovasc Res. 2006;69:614-24. https://doi.org/10.1016/j.cardiores.2005.08.002
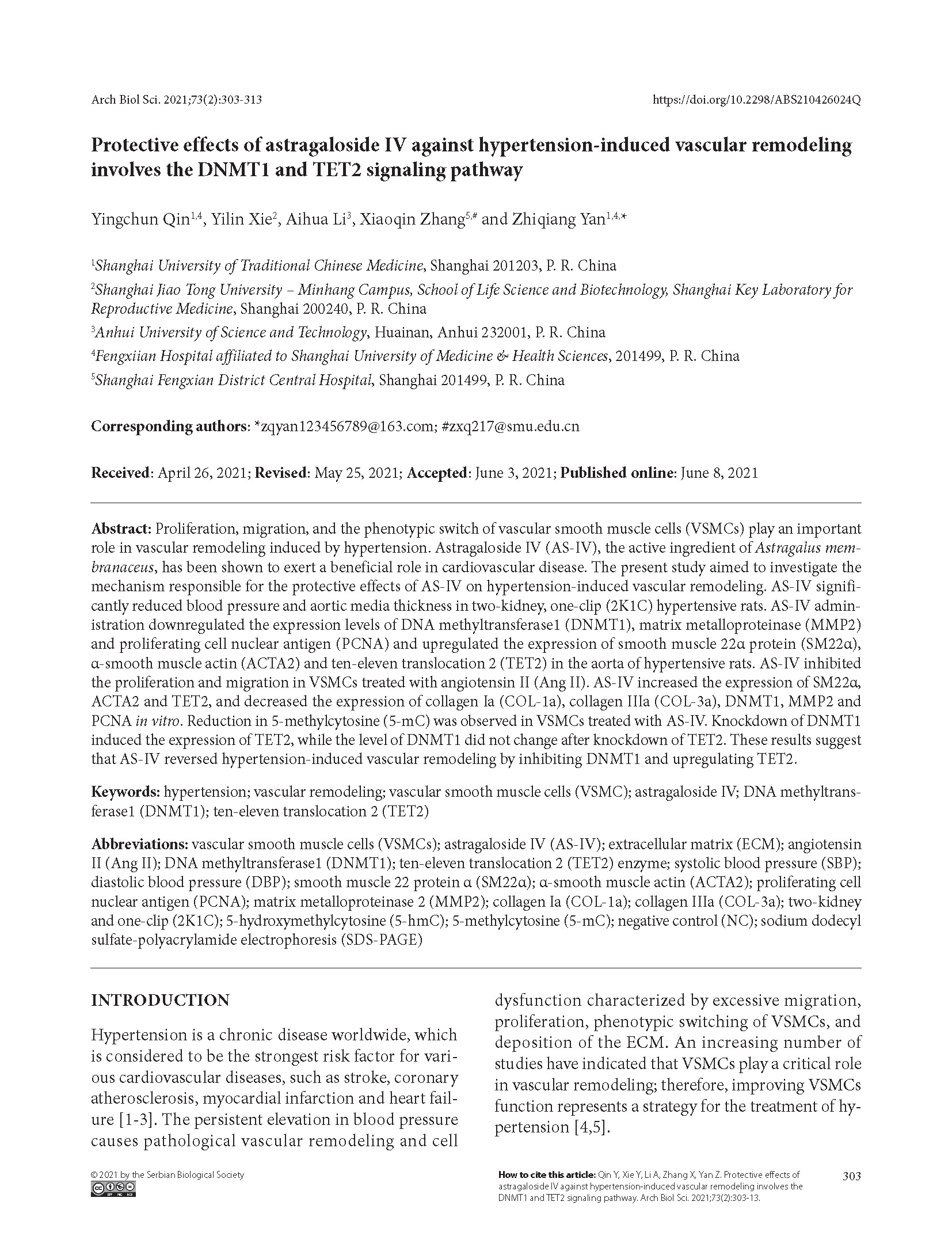
Downloads
Published
How to Cite
Issue
Section
License
Copyright (c) 2021 Archives of Biological Sciences

This work is licensed under a Creative Commons Attribution-NonCommercial-NoDerivatives 4.0 International License.
Authors grant the journal right of first publication with the work simultaneously licensed under a Creative Commons Attribution 4.0 International License that allows others to share the work with an acknowledgment of the work’s authorship and initial publication in this journal.